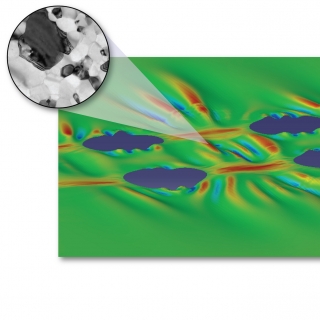
Mechanics and Physics of Disordered and Non-equilibrium Materials
Our research seeks to exploit the nonequilibrium nature of glassy materials, such as metallic glasses, as a means of controlling mechanical behavior. We develop synthesis, processing and testing methods to extend the control of the structural state of glasses well beyond the reach of traditional bulk treatments, ultimately defining the limits of the structural state spectrum - from ultrastable (kinetically stable) to liquid-like. In doing so, the range of mechanical behavior that can be obtained via isochemical treatments of glasses, including the limits of strength and damage tolerance as well as the critical point at which a brittle glass transitions to a ductile one occurs. We hypothesize that our approach will enable not only the formulation of structural state - property relationships in glasses, but also an unprecedented control over their mechanical properties. An overarching goal of this project is to synthesize strong, tough, and kinetically stable glasses and provide design rules for other nonequilibrium systems where structural integrity is the predominant performance criterion.
We also study nanocrystalline metals and alloys, which are appealing for structural applications owing to a suite of excellent mechanical properties. The large fraction of interfacial material delineating neighboring crystallites is, however, responsible for both high strength as well as microstructural instability and poor damage tolerance. While the incorporation of solute elements that tend to segregate to grain boundaries can have a stabilizing effect due to thermodynamic or kinetic factors, the application of mechanical stresses can still lead to grain growth in otherwise thermally stable nanocrystalline materials. We hypothesize that control of grain boundary chemistry in nanocrystalline alloys can be used to tailor the thermal and mechanical stability of nanocrystalline materials against grain boundary migration under normal service conditions. In cases where extreme mechanical environments are encountered (e.g. at stress concentrations such as crack tips), stress-driven grain boundary migration can be triggered to respond to damage evolution, with the threshold stress set by the dopants residing at grain boundaries. This approach shows great promise for new nanocrystalline materials with combinations of high strength and unprecedented damage tolerance. We develop new approaches to the synthesis, characterization, and mechanistic understanding of nanocrystalline metals with both high strength and robust damage tolerance by programming the effect of mechanical stress on grain boundary migration via interfacial doping.
Our vision is to inform novel synthesis strategies and provide alloy design rules to control the extent of disorder in glassy and interface-mediated materials.